A Single-Center Experience of Robotic-Assisted Spine Surgery in Korea : Analysis of Screw Accuracy, Potential Risk Factor of Screw Malposition and Learning Curve
Article information
Abstract
Objective
Recently, robotic-assisted spine surgery (RASS) has been considered a minimally invasive and relatively accurate method. In total, 495 robotic-assisted pedicle screw fixation (RAPSF) procedures were attempted on 100 patients during a 14-month period. The current study aimed to analyze the accuracy, potential risk factors, and learning curve of RAPSF.
Methods
This retrospective study evaluated the position of RAPSF using the Gertzbein and Robbins scale (GRS). The accuracy was analyzed using the ratio of the clinically acceptable group (GRS grades A and B), the dissatisfying group (GRS grades C, D, and E), and the Surgical Evaluation Assistant program. The RAPSF was divided into the no-breached group (GRS grade A) and breached group (GRS grades B, C, D, and E), and the potential risk factors of RAPSF were evaluated. The learning curve was analyzed by changes in robot-used time per screw and the occurrence tendency of breached and failed screws according to case accumulation.
Results
The clinically acceptable group in RAPSF was 98.12%. In the analysis using the Surgical Evaluation Assistant program, the tip offset was 2.37±1.89 mm, the tail offset was 3.09±1.90 mm, and the angular offset was 3.72°±2.72°. In the analysis of potential risk factors, the difference in screw fixation level (p=0.009) and segmental distance between the tracker and the instrumented level (p=0.001) between the no-breached and breached group were statistically significant, but not for the other factors. The mean difference between the no-breach and breach groups was statistically significant in terms of pedicle width (p<0.001) and tail offset (p=0.042). In the learning curve analysis, the occurrence of breached and failed screws and the robot-used time per screw screws showed a significant decreasing trend.
Conclusion
In the current study, RAPSF was highly accurate and the specific potential risk factors were not identified. However, pedicle width was presumed to be related to breached screw. Meanwhile, the robot-used time per screw and the incidence of breached and failed screws decreased with the learning curve.
INTRODUCTION
The fourth industrial revolution is leading the healthcare industry to a wind of change that has never been experienced before [6,25]. Accordingly, the development of surgical techniques has progressed by leaps and bounds for decades, and the equipment required for surgery has also rapidly developed. The use of da Vinci Surgical System (Intuitive Surgical Inc., Sunnyvale, CA, USA) as a robotic surgical device was approved by the U.S. Food and Drug Administration in 2000, and it opened the era of robotic surgery. Moreover, medical robots are currently applied to various surgeries [11]. Robotic surgery had negative rather than positive evaluation results in its early introduction [4]. However, numerous clinical data have been collected over the last 10 years, and robotic surgery has progressively proven to be more clinically effective than conventional methods. Thus, the medical paradigm is also gradually changing [12,18,23]. This trend was reflected in spine surgery without exception, and robotic-assisted pedicle screw fixation (RAPSF) emerged in the field of spine surgery. In 2004, a robotic system was applied to pedicle screw fixation, and Spine Assist (Mazor Robotics Ltd., Caesarea, Israel), the first spine robot, was developed [8]. Thereafter, the robot has been further developed and improved to date. In addition to Mazor, Globus’ EXCELSIUS (Globus Medical Inc., Audubon, PA, USA) and Medtech’s ROSA SPINE (Medtech, Montpellier, France) have been developed for pedicle screw fixation and are used worldwide with the approval of the U.S. Food and Drug Administration [8,14]. Our hospital was selected for the medical surgical robot project, part of the 2021 service robot utilization demonstration project. As a result, Curexo’s CUVIS-spine robot system (CUREXO, Seoul, Korea), developed with domestic technology, was installed. Since applying the first pedicle screw fixation with CUVIS-spine robotic system in October 2021, RAPSF has been used in 100 patients by November 2022. CUVIS-spine is expected to show similar performance to the previously developed spine robot, but there has been no domestic research on this yet. Therefore, in the current study, based on the data collected from 100 patients who attempted RAPSF, the accuracy of pedicle screw placement, potential risk factors, and learning curve were analyzed and want to share experience.
MATERIALS AND METHODS
This retrospective study was approved by the Institutional Review Board of Pusan National University Yangsan Hospital (IRB 05-2023-118).
Demographic characteristics of the patients
The data from attempted 495 RAPSF were analyzed retrospectively (Fig. 1). Relevant data were obtained from the hospital’s electronic medical records and the picture archiving and communication system. The inclusion criteria included patients who diagnosed with herniated intervertebral disc with segmental instability, severe spinal stenosis (grade 3-4), spondylolisthesis (grade 1-2), and scoliosis, those who ineffective conservative treatment for 6 months. The patients underwent computed tomography (CT) scans pre- and postoperatively, and the patient’s bone mineral density (BMD) and body mass index (BMI) were examined before surgery (Table 1). All the patients underwent interbody fusion (oblique lateral interbody fusion [OLIF] or OLIF + posterior lumbar interbody fusion), of which nine patients had decompressive laminectomy. In addition, all RAPSF were conducted with percutaneous fixation using CUVIS-spine. Patients with spinal tumors, spinal tuberculosis, and severe spinal deformities were excluded from the analysis.
Robotic-assisted pedicle screw fixation
CUVIS-spine comprises a main console with an optical camera, a floor-mounted robot arm, and a staff console (Fig. 2A). The robotic system supports a scan and plan method, considering intraoperative CT scans based on the acquired images. Therefore, the first step was an intraoperative CT scan with an O-arm on the target vertebrae. The images of all patients were taken with a tracker that fixed the two-level upper spinous process of the upper instrumented vertebrae (Fig. 2B). The second step was the planning and previewing of the target vertebrae. Once screw trajectories were planned on the workstation, all screw plans could be previewed. In that preview, the screw trajectory and rod alignment could be rechecked (Fig. 2C). The third step was the trajectory fixation and skin incision. Once the robot arm was fixed to the planned trajectory, a skin incision was made. At this time, the skin and fascia should be released sufficiently to prevent trajectory shifting (Fig. 2D and E). The fourth step was the insertion of the expander to create a working corridor and drilling point (Fig. 2F). Prior to drilling, the insertion point was double-checked using a ball-tip probe, whether the drill point was missed or consistent with the existing plan (Fig. 2G). The fifth step was drilling the entry point and completing the tapping. After drilling and tapping, the trajectory was rechecked at each step with a ball-tip probe (Fig. 2H). Finally, the screw was inserted with the assistance of the robot arm (Fig. 2I).

A : Component of CUVIS-spine. B : Intraoperative O-arm scan. C : Planning and preview. D : Trajectory fixation and skin incision. E : Releasing the skin and fascia. F : Insertion of the expander. G : Drilling point confirmation using a ball-tip probe. H : After drilling and tapping, checking again with a ball-tip probe. I : Screw insertion under robotic guidance.
Evaluation of screw placement accuracy
After screw insertions were completed in each patient, a postoperative CT scan was performed using an O-arm to evaluate screw placement. The position of RAPSF was evaluated by the Gertzbein and Robbins scale (GRS) [34]. The grading system reflects the deviation of the screw from intrapedicular trajectory. Grade A, perfectly within the pedicle; grade B, pedicle cortical breach <2 mm; grade C, pedicle cortical breach ≥2 mm and <4 mm; grade D, pedicle cortical breach ≥4 mm and <6 mm; grade E, pedicle cortical breach ≥6 mm. Accuracy was assessed thru the ratio of the clinically acceptable group (GRS grades A and B) and the dissatisfying group (GRS grades C, D, and E) [9]. Another method for accuracy analysis using the Surgical Evaluation Assistant (SEA) program provided by the manufacturer of CUVIS-spine. Using the SEA program, intraoperative and postoperative CT scan images could be merged, and the bias between the actual and planned screw path could be measured. The fiducial registration error (FRE), tip offset, tail offset, and angular offset were evaluated for the bias analysis (Fig. 3). FRE was defined as the precision of merging between intra- and postoperative CT scan images, with a value of ≤1 mm indicating better merging. Tip offset was defined as the error of the tip point of the inserted screw compared with the planned one. Tail offset was defined as the error of the entry point of the inserted screw compared with the planned one. Finally, the angular offset was defined as the angular error of the inserted screw path compared with the planned one.
Analysis of potential risk factor of screw malposition
The RAPSF was divided into the no-breached group (GRS grade A) and the breached group (GRS grades B, C, D, and E). Based on previous studies [33,36-38], the potential risk factors were classified and analyzed as follows : 1) age (≥70 and <70 years old), 2) sex (male, female), 3) BMI (non-obese group, <25 kg/m2; overweight group, 25 kg/m2 ≤BMI<30 kg/m2; and obese group, ≥30 kg/m2), 4) BMD measured using dual-energy X-ray absorptiometry at the lumbar region (non-osteopenia group, T value of >-1.0; the below osteopenia group, T value of ≤-1.0), 5) left and right screw side, 6) screw fixation level (L2, L3, L4, L5, and S1), 7) segmental distance (SD) between the tracker and the instrumented level (Fig. 4 [two, three, four, and five levels]), and 8) preoperative diagnosis (spondylolisthesis, herniated intervertebral disc [HIVD], spinal stenosis, and scoliosis). In addition, the mean difference in BMI, BMD, pedicle width and height, and SEA analysis results between the no-breached and breached groups was evaluated.
Assessment of learning curve
The time interval between the intraoperative O-arm scan taken for planning and the postoperative O-arm scan for validating screw placement was considered the time using the robot. Two methods were used to analyze the learning curve. One was a method to examine the occurrence tendency of breached and failed screws according to the accumulation of RAPSF. The other was to investigate the cumulative change in robot-used time per screw, measured by dividing the time using the robot by the number of screws inserted per patient.
Statistical analysis
Analyses were performed using the Statistical Package for the Social Sciences software version 26.0 (IBM Co., Armonk, NY, USA) and MedCalc version 19.6 (MedCalc Software Ltd., Ostend, Belgium). Continuous variables with normal distribution were expressed as means with standard deviations. Categorical variables were expressed as a quantity. Categorical data were analyzed using Fisher’s exact test and the chi-square test. The Student’s t-test and one-way analysis of variance were used to compare independent data with normal distribution. Learning curves were examined using the binomial logistic regression (Figs. 5 and 6). A p-value of <0.05 was considered statistically significant.

Curve for changes in the breached + failed screw probability. The curve converged to no-breached with the accumulation of cases. CI : confidence interval.
RESULTS
In this study, RAPSF was attempted at 495 on 100 patients. In total, 479 screws were inserted and 16 failed screws. In the case of failed screws, the risk was high due to a significant deviation during the actual procedure compared with the planned one. Hence, all failed procedures were converted to fixation with a C-arm guide. Based on the GRS classification, 412 screws were grade A; 58, grade B; seven, grade C; one, grade D; and one, grade E. Rescue fixation was not performed on nine screws below grade C as the breach did not indicate the need for repositioning (Table 2).
Screw placement accuracy
Of the 479 RAPSFs, excluding failed screws, the clinically acceptable group was 98.12% (470/479). With the SEA program, the offsets of 442 RAPSFs, except for 37 screws in which the original planning file was missing and failed screws, were measured. The FRE was 0.64±0.35 mm, the tip offset was 2.37 ±1.89 mm, the tail offset was 3.09±1.90 mm, and the angular offset was 3.72°±2.72° (Table 3).
Potential risk factor of screw malposition
The potential risk factors of 479 RAPSFs, excluding failed screws, were analyzed. There was no significant difference in terms of age (p=0.286), sex (p=0.223), BMI (p=0.751), BMD (p=0.420), screw side (p=0.539), spondylolisthesis (p=0.546), HIVD (p=0.408), spinal stenosis (p=0.297), and scoliosis (p=0.476) between the no-breached and breached group. However, the difference in screw fixation level (p=0.009) and SD between the tracker and the instrumented level (p=0.010) between the no-breached and breached group were statistically significant. In addition, the breached group was significantly related to screw fixation at the upper level and instrumented levels closer to the tracker (Table 4). In the mean difference analysis, the two groups did not significantly differ in terms of BMI and BMD. However, the mean difference between the no-breached and breached group was statistically significant in terms of pedicle width (p<0.001) and tail offset (p=0.042) (Table 5).
Learning curve
The mean robot-used time in 100 patients was 114.13±53.02 minutes, and the mean robot-used time per screw was 21.04±8.30 minutes. The occurrence tendency of breached and failed screws was significantly decreased with the accumulation of cases (p=0.008), and the curve converged to no-breached (Fig. 5). Further, the robot-used time per screw significantly decreased and stabilized with the accumulation of cases (Fig. 6).
DISCUSSION
Pedicle screw fixation is an important procedure for maintaining the mechanical stability of the spine and is widely used in several types of spine surgeries [7,24]. The dissatisfaction rates were 10–20% in the conventional free-hand pedicle screw fixation and 5–10% in C-arm-guided pedicle screw fixation [10,19,20,26,28,30]. Pedicle screw fixation is important for the success of spinal fusion. Thus, spine surgeons are always vigilant about the accuracy of pedicle screw placement to prevent related complications. RASS is a minimally invasive surgery used in pedicle screw fixation and is of major interest in recent spine surgery [35]. From this point of view, the accuracy and potential risk factors of RAPSF are also clinically important areas of interest. To date, there are several international reports on the accuracy and risk factors of RAPSF. However, the number of domestic studies was limited. Since CUVIS-spine, the first spinal surgery robot developed in Korea was installed in our hospital and performed RASS, it was thought necessary to evaluate the accuracy, potential risk factors, and learning curve of RASS based on the accumulated data.
Generally, RASS can provide three-dimensional imaging and precision guidance and eliminate possible human error, such as tremors and fatigue, which can occur in conventional surgeries [1]. Moreover, real-time imaging and navigation technology can be used to improve accuracy, reduce complications, and enhance patient safety. Previous studies have shown that RAPSF has a high accuracy rate, ranging from 95% to 98% [20,28]. A similar accuracy was observed in this study. In the analysis, failed screws were excluded. However, even if they were included, the accuracy was 94.94% (470/495), similar to previous reports.
The analysis of potential risk factors in this study differed somewhat from that in the published literature [21,37,38]. According to a recently published literature by Zhang et al. [37], severe obesity was considered a potential risk factor of unsatisfactory screw. However, in this study, BMI was not analyzed as the potential risk factor, and the Student’s t-test on BMI between the two groups yielded results that could be interpreted differently from those of previous reports. Although not statistically significant in the analysis, the no-breached group had a higher BMI than the breached group. These results were thought to be attributed to differences in robotic systems. Since the robot system using K-wire proceeds with minimal skin incision, the excessive soft tissue in obese patients may exert pressure on the screw trajectory, reducing accuracy. However, in the case of CUVIS-spine, the pressure of the surrounding soft tissue does not have a significant effect on the deviation because sufficient soft tissue releasing was achieved through muscle and fascia incision after confirming the target point without using K-wire.
Zhang et al. [38] analyzed the increased distance between the instrumented level and the patient tracker as a significant risk factor and reported the gap distance that exceeded three levels from the tracker as a potential risk factor for screw displacement. However, in this analysis, the rather breached group was related to the closeness between the instrumented level and the tracker. In other words, it can be inferred that the instrumented level and the proximity of the tracker correspond to the upper-level screw fixation in the surgery, which may be related to the breached screws. In addition, the breached group was significantly associated with screw fixation at the upper vertebral level. Based on these two results, it was thought that the breached screws were related to some other factor affecting upper vertebrae level screw fixation rather than the distance between the tracker and the instrumented level. Furthermore, in this study, there was a statistically significant mean difference in pedicle width in the breached group, and it was confirmed that the pedicle width gradually narrowed toward the upper vertebrae spine (Fig. 7). Additionally, there are several reports on pedicle size and screw placement safety [2,22,32]. Thus, considering all of the above, pedicle width seems to be related to breached screws, and it is unlikely that screw fixation level and SD between the tracker and the instrumented level will be considered independent potential risk factors for RAPSF.

One-way analysis of variance of the average difference in pedicle width at each level. The upper level showed that the mean of the pedicle screw width significantly narrower.
Analysis of the mean difference between the two groups of SEA data identified a significant difference in the tail offset. The exact cause of this difference has not yet been analyzed. Nonetheless, a comprehensive review of specific failed screw cases identified the possible causes of tail offset. The first factor was focal sclerosis of the pedicle entry surface at the point of screw insertion. In the SEA program, the orange color is the planning path, and the blue color is the real screw position (Fig. 8). In this case, skiving of the right S1 screw occurred inferior to the pedicle entry surface. On a preoperative CT scan, the Hounsfield unit (HU) of the right S1 was approximately 700–800, which was higher than an HU of 300–500 in other areas. Even if BMD is normal, it was assumed that it skived to the inferior side because sufficient drilling at the entry point was difficult due to focal sclerosis. The second factor was the focal anatomy. In this case, the right L4 screw was skiving to the lateral side. Typically, the entry point was selected at the blunt surface of the pedicle. However, in few cases, the entry point was changed to a sharp surface while adjusting the rod contour (Fig. 9). A sharp entry point could cause skiving and how to overcome it have been mentioned in previous reports [17]. Therefore, it is important to select an appropriate entry point that is not sharp to prevent skiving.
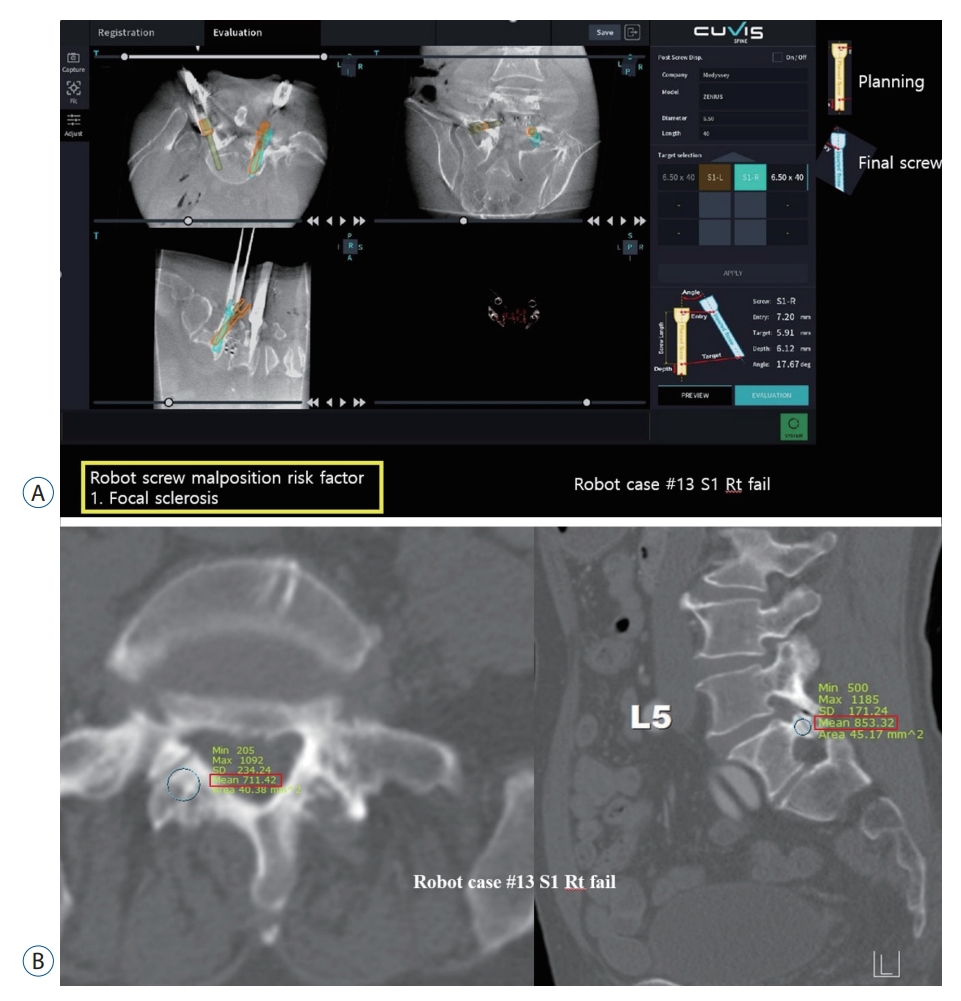
A : Entry deviation caused by focal sclerosis. B : The entry point had a high Hounsfield unit value. Rt : right.

A : Entry deviation caused by the focal anatomy (sharp entry point). B : Change to a sharp entry point after rod alignment contour. Rt : right.
The learning curve for RASS involves both technical proficiency with the robotic system and adaptability to changes in surgical techniques. Spine surgeons must have the necessary skills to operate robotic systems accurately and safely and may require additional training or hands-on experience. Spine surgeons should also learn to integrate robotic systems into their surgical workflow to optimize outcomes. The learning curve depends on various factors, including the surgeon’s previous experience with robotic surgery, the complexity of the surgical procedure, and the specific robotic system used. In general, several surgical experiences may be required before the spine surgeon becomes accustomed to performing RASS independently. Based on these factors, several studies have reported the learning curve of RASS [13,16,27]. In previous reports, the learning curve of RASS was shown after 3–30 surgeries or 15– 60 screw fixations. In terms of operative time, the learning curve showed an average reduction of 6 minutes per month in 3–10 RASS [3,5,15,29,31]. In the binomial logistic regression analysis, the initial value was lower than the cutoff value (0.5) due to the high accuracy of RAPSF. The occurrence of breached screws, including failed screws, was mostly observed in the initial process of 100 RAPSF, and it showed a curve that significantly converged to no-breached with the accumulation of cases. The sporadic focal increase in robot-used time per screw occurring between 40 and 60 operations was frequently caused by delays in intraoperative CT scan image transmission to the robotic system or additional scans due to inappropriate O-arm scans in the robotic system, rather than specific difficult situations in screw fixation. Considering these aspects, the robot-used time per screw fixation decreased significantly as the number of operations accumulated, and the learning curve was shown after the number of surgeries exceeded 20–30.
Thus far, the results of this study and the outcomes of RASS are noteworthy. Nevertheless, the procedure has several disadvantages. It is generally more expensive than the conventional methods, requires a long operation time, and not all patients are suitable candidates. There is also a risk of technical malfunction. However, it also has evident advantages. First, since robot-related technology development is currently in progress, the possibility of improving accuracy and operation-related manuals is endless. Second, radiation-related complications can be lowered by reducing the time the patient and surgeon are exposed to radiation. In addition, the robot can be useful when the pedicle is not well identified in the C-arm or when high accuracy is required for screw re-insertion during revision surgery. One of the significant advantages of RASS is its ability to achieve greater precision and accuracy and to minimize the risks of complications among inexperienced spine surgeons.
The current study had several limitations. First, as a single-center retrospective study, detailed GRS ratings could not be expressed as learning curves because the sample size was not large. Second, in addition to the potential risk factors mentioned in the analysis, other factors, such as spinal deformity, the movement of the thorax according to the patient’s breathing volume, and concordance rate during intraoperative CT scan and patient synchronization, which could affect accuracy, were not included. Third, RAPSF of the cervical and thoracic spine was not included in this analysis because it is not supported by the CUVIS-spine system. Nevertheless, to the best of knowledge, this study first reported the potential risk factors and learning curve of domestically developed spine surgery robots, and the analysis result is described in detail. We believe that this study can provide information that can be a cornerstone for other surgeons who are starting to use RASS.
CONCLUSION
In the current study, RAPSF was highly accurate, and although the screw fixation level and SD between the tracker and the instrumented level were statistically significant, it was difficult to consider them as independent potential risk factors, and the specific potential risk factors were not identified. However, it is presumed that the pedicle width is related to breached screws as in the conventional screw fixation method. In addition, focal sclerosis or the sharp surface of the pedicle entry point may cause skiving. In the analysis, breached screws commonly occurred at the early stage of RAPSF, after which the robot-used time per screw and the occurrence of breached and failed screws decreased according to the learning curve. RASS still has some limitations. However, it is expected to be able to provide guidance to inexperienced spine surgeons and ensure patient safety.
Notes
Conflicts of interest
Dong Wuk Son has been editorial board of JKNS since November 2017. He was not involved in the review process of this original article. No potential conflict of interest relevant to this article was reported.
Informed consent
This type of study does not require informed consent.
Author contributions
Conceptualization : BKO, DWS; Data curation : BKO, JSL; Formal analysis : BKO, SHL; Funding acquisition : BKO, YHK, SKS; Methodology : BKO, SWL, GSS; Project administration : BKO, DWS, SY; Visualization : BKO; Writing - original draft : BKO, DWS; Writing - review & editing : GSS, SWL
Data sharing
None
Preprint
None
Acknowledgements
This work was supported by the National Research Foundation of Korea Grant funded by the Korea Government (NRF-2021R1G1A1011491). The sponsor played no role in the study design, data collection, or analysis, or decision to submit the article for publication.